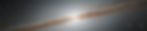
PhD Positions in gas accretion and galactic winds
​
Embedding: This project is part of the ERC Advance Grant project "FLOWS": Gas flows in and out of galaxies: solving the cosmic baryon cycle
​
Group: The FLOWS group will consist of 3 PhD students, 2 postdocs and the PI
​
PI: Prof. F. Fraternali
Collaborators: Prof. K. Rubin (San Diego State University, USA) and Prof. F. Marinacci (University of Bologna, Italy)
Scientific background
The majority of matter in the Universe is in the form of gas, found in galaxies and their surrounding environments. Gas flows, including accretion and ejection, play a crucial role in the growth, star formation, and overall properties of galaxies. However, our understanding of these gas flows is limited. Observationally, we have gathered a significant amount of data on gas in and outside of galaxies, but the interpretation is uncertain. Theoretical models also lack a clear understanding of how large-scale gas flows relate to gas accretion onto galaxies and the effectiveness of ejective feedback in removing gas. This project aims to address these gaps in knowledge by investigating all gas flows and incorporating observations into a coherent physical picture.
​
PhD 1. Dissecting galactic winds​
Galactic winds, powered by supernova explosions and active galactic nuclei, are thought to play critical roles in shaping several properties of galaxies, including their stellar mass and scaling relations. However, the properties of the gas outflows produced by galactic winds remain largely undetermined and the theoretical models poorly constrained. This PhD project aims to determine these properties, such as the mass loading factor, with a new methodology applied to emission-line observations of both ionised and neutral gas over cosmic time.
​
Previous research in this field has primarily relied on fitting individual line profiles with multiple Gaussian components or selecting specific regions in 2D kinematic maps. Our approach, instead, will be groundbreaking as we intend to develop 6D models of biconical galactic winds (comprising of 3 positions and 3 velocities). These models will then be projected along a line of sight to generate 3D mock emission-line datacubes (Fig. 2), which can be directly compared with actual data using an MCMC method and our dedicated computer cluster. By utilizing the information contained within the datacubes, we will be able to differentiate between rotating discs and winds, and simultaneously fit both a wind and a rotating disc, which is not feasible using 1D or 2D methods.
Initially, we will apply our 3D software to datacubes obtained from nearby starburst galaxies, utilizing ionised gas as well as cooler tracers. These datacubes will be sourced from studies such as Reichardt Chu et al. (2022). Concurrently, we will also utilize publicly available hydrodynamical cosmological simulations (e.g., Wetzel et al. 2022) and isolated-galaxy simulations (e.g., Marinacci et al. 2019) to construct mock datacubes before applying our software. Subsequently, our focus will shift towards high-redshift main-sequence galaxies, where we will analyse optical rest-frame emission-line data (specifically Ha and [OIII]) as well as ALMA data. Numerous observations are already present in archives. Additionally, forthcoming data from the JWST and ERIS will also be incorporated.
​
Plan of the work.
Year 1: Develop a 3D modelling software to study galactic winds and test it on mock datacubes from idealised hydrodynamical simulations and on nearby starburst galaxies (1 paper).
Year 2: Expand the 3D software including tests on cosmological simulations from which we will generate mock data cubes.
Year 3: Transition to high-z main-sequence galaxies and apply the 3D software to optical rest-frame emission-line data, including Ha and [OIII], as well as ALMA data (2 papers).
Year 4: Consolidate findings and release the 3D modeling software to the scientific community; provide outflow rate measurements for local starburst and high-z galaxies (1 paper).

Figure 1. 3D rendering of an emission-line (HI) datacube and a rotating-disc model obtained with 3DBarolo that has been fitted to the data. The green emission, not reproduced by the model, is outflowing gas. This type of emission will be reproduced by our new software developed by in PhD project.
PhD 2. Unveiling Gas Accretion onto Galaxies
​
The primary goal of this PhD project is to unveil and measure, for the first time, gas accretion via fountain accretion in local and high-redshift galaxies. This will be achieved by developing a new dynamical model of the galactic fountain, informed by ultra-high-resolution magneto-hydrodynamical simulations, and fitting it directly to emission-line datacubes.
We will start with the "extragas" code from previous studies (Fraternali & Binney 2008; Li et al. 2022), which produces 3D mock observations from a fountain accretion model by accounting for the galactic potential and hydrodynamical effects. The models have a few free parameters, such as ejection velocities of the fountain gas and the coronal condensation rate, that can be estimated by comparing with observations. The key observable is the gas kinematics at the disc-halo interface, specifically in the extraplanar gas layer. The presence of coronal condensation modifies fountain gas trajectories, providing a detectable signature.
​
We will enhance the extragas software by implementing a Markov Chain Monte Carlo (MCMC) algorithm for parameter estimation and improving the hydrodynamical interactions between fountain clouds and hot gas. This improvement will utilize a library of approximately 100 ultra-high-resolution magneto-hydrodynamical simulations (Fig. 2), exploring a wide parameter space. At least half of these simulations will be run on our in-house computer cluster. The simulations will offer valuable data on condensation rates under different fountain and corona conditions, contributing to the development of the first simulation-informed analytic model (SIAM) for a galactic fountain.
​
The project will apply this model to observations of local galaxies, focusing on H I and Hα datacubes. Abundant data from current surveys and upcoming observations from SKA pathfinders will be available. Our goals include determining the prevalence of fountain condensation in nearby galaxies, assessing gas accretion profiles, and analyzing the angular momentum of the accreting gas. We will also use ionized gas as a tracer for accretion, utilizing observations from the William Herschel Telescope's Enhanced Area Velocity Explorer (WEAVE). For higher redshifts, we will employ data from the Very Large Telescope (VLT) and the Atacama Large Millimeter/submillimeter Array (ALMA), particularly [C II] observations that reveal extraplanar gas layers in galaxies up to z ≈ 4.5.
​
-
Year 1: Modify the "extragas" code by implementing an MCMC algorithm.
-
Year 2: Develop a library of ultra-high-resolution magneto-hydrodynamical simulations.
-
Year 3: Apply the improved model to observational data of local galaxies to measure gas accretion rates and profiles.
-
Year 4: Extend the analysis to high-redshift galaxies to construct a coherent picture of gas accretion onto galaxies.

Figure 2. Temperature slice of a magneto-hydrodynamical simulation of a cloud moving (along x) through the hot CGM. The magnetic field is initially perpendicular to the motion and the simulation also includes radiative cooling and anisotropic thermal conduction. All gas below 20000 K has been coloured dark blue, gas above a million degree is in red. From Kooij et al. (2021).
To apply for one or both positions go to:
Bibliography
​
Borthakur, S., Heckman, T., Tumlinson, J., Bordoloi, R., Thom, C., Catinella, B., Schiminovich, D., Davé, R., Kauffmann, G., Moran, S. M., and Saintonge, A. 2015, ApJ, 813, 46
Fraternali, F. & Binney, J.J. 2008, MNRAS, 386, 935
Kooij, R., Grønnow, A., and Fraternali, F. 2021, MNRAS, 502, 1263
Marinacci, F., Sales, L. V., Vogelsberger, M., Torrey, P., and Springel, V. 2019, MNRAS, 489, 4233
Reichardt Chu, B., Fisher, D. B., Nielsen, N. M., Chisholm, J., Girard, M., Kacprzak, G. G., Bolatto, A., Herrera-Camus, R., Sandstrom, K., Li, M., et al. 2022, MNRAS, 511, 5782
Li, A., Fraternali, F., Marasco, A., Trager, S.C., Pezzulli, G., Mancera Piña, P.E., Verheijen, M.A.W. 2023, MNRAS, 520, 147
Wetzel, A., Hayward, C. C., Sanderson, R. E., Ma, X., Angles-Alcazar, D., Feldmann, R., Chan, T. K., El-Badry, K., Wheeler, C., Garrison-Kimmel, S., Nikakhtar, F., et al. P. F. 2022
Wilde, M. C., Werk, J. K., Burchett, J. N., Prochaska, J. X., Tchernyshyov, K., Tripp, T. M., Tejos, N., Lehner, N., Bordoloi, R., O'Meara, J. M., and Tumlinson, J. 2021, ApJ, 912, 9